Helion’s MTF vs. Other Fusion Technologies: A Comparative Case Study
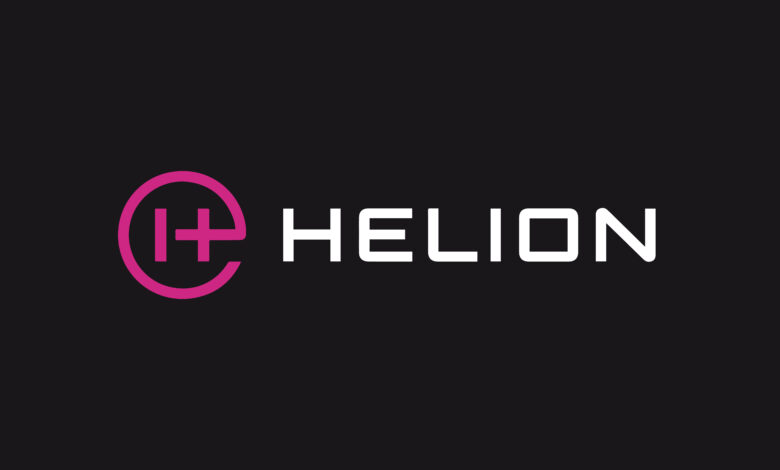
In the rapidly evolving field of nuclear fusion, Helion Energy has emerged as a transformative force, challenging long-held assumptions about what is required to achieve practical and scalable fusion energy. Unlike traditional approaches that rely on magnetic confinement (such as tokamaks) or inertial confinement (such as laser-driven fusion), Helion employs a distinctive Magnetized Target Fusion (MTF) method—a hybrid that combines the best aspects of magnetic and inertial techniques.
The significance of Helion’s design lies in its pulsed operational mode, linear reactor geometry, and direct electricity conversion, all while using an aneutronic fuel mix of deuterium and helium-3. This fusion pathway produces charged particles instead of high-energy neutrons, thus drastically reducing radioactive waste and enabling the direct capture of electrical energy without the need for inefficient steam turbines.
Helion’s sixth prototype, Trenta, achieved the critical milestone of 100 million °C—widely considered the threshold for fusion. Its seventh prototype, Polaris, operational in 2024, is designed to validate Helion’s claim to net electricity generation. Perhaps most notably, Helion became the first fusion company in history to sign a commercial power purchase agreement (PPA)—a deal to deliver 50 MW of electricity to Microsoft by 2028.
This case study dissects Helion’s strategy and technological choices in depth, offering a side-by-side comparison with other leading fusion efforts, including ITER, Commonwealth Fusion Systems (CFS), TAE Technologies, General Fusion, and National Ignition Facility (NIF). Each represents a fundamentally different take on fusion—from toroidal magnetic confinement and ultra-dense laser compression to mechanical liquid-liner implosions and advanced beam-driven plasmas.
We analyze:
- Helion’s unique physics and engineering model
- Advantages of direct energy capture and aneutronic fuel.
- Timelines and commercialization strategies
- Major technical risks and their mitigation
- Global investment and partnerships
-
A 2035-outlook on potential fusion winners
By comparing real-world milestones, financial backing, and scientific hurdles, this white paper aims to clarify the true status of fusion energy commercialization and why Helion’s MTF pathway may offer the shortest path to grid-scale fusion electricity.
The Promise and the Puzzle of Fusion Energy
2.1 The Holy Grail of Clean Power
Nuclear fusion is often described as the “holy grail” of energy production—a near-limitless, zero-carbon power source that mimics the process powering our Sun. It promises a solution to the energy crisis without the radioactive waste or meltdown risks associated with nuclear fission. A single glass of fusion fuel—deuterium and helium-3—could, in theory, power an entire city for a day.
But the practical challenges of achieving fusion are formidable. The process demands temperatures exceeding 100 million °C, ultra-precise plasma confinement, and the ability to extract more energy from the reaction than is used to initiate it. For decades, these hurdles left fusion confined to academic theory and government-funded experiments.
2.2 From Big Science to Private Acceleration
Historically, progress in fusion was driven by large public-sector efforts, especially the ITER (International Thermonuclear Experimental Reactor) project—a $40+ billion collaboration between 35 nations aimed at building the world’s largest tokamak. But ITER’s scale and complexity have led to major delays, with net fusion power now expected only by the mid-2030s.
By contrast, the past ten years have witnessed a private fusion renaissance. Agile startups, empowered by advancements in AI, superconducting magnets, and materials science, have entered the scene with new reactor geometries, novel fuels, and aggressive commercialization timelines. These include Helion Energy, Commonwealth Fusion Systems, TAE Technologies, and General Fusion, each with its own engineering ethos and vision for the future of fusion.
2.3 The Stakes
At stake is not just scientific glory, but the multi-trillion-dollar energy market. If even one private company can deliver a grid-connected fusion reactor within the next decade, it could transform global power generation, drastically cut carbon emissions, and render many fossil-fuel-based systems obsolete.
Among this new generation of innovators, Helion Energy has drawn special attention—not only for its groundbreaking Magnetized Target Fusion approach, but also for its strong financial backing and actual customer agreements.
Helion Energy’s Magnetized Target Fusion (MTF) Approach
Helion’s fusion system is conceptually elegant yet technically bold. It employs a magneto-inertial confinement strategy, combining key advantages from both magnetic and inertial fusion while sidestepping their most intractable problems.
3.1 Field-Reversed Configurations and Pulsed Operation
At the core of Helion’s device are Field-Reversed Configurations (FRCs)—donut-shaped plasma structures confined by their own internal magnetic fields. These FRCs are formed at opposite ends of a linear accelerator-style reactor and launched toward one another at high speed.
When the FRCs collide in the center of the device, external magnetic coils deliver a rapid compression pulse, squeezing the plasma to fusion conditions. This pulsed approach, repeating many times per second, is fundamentally different from the continuous operation of tokamaks, which strive for a steady-state plasma over long durations.
“It’s like firing mini fusion bullets, one after the other, rather than trying to keep a fire constantly burning.” — David Kirtley, Helion CEO
This high-frequency repetition aims to deliver near-continuous power, while offering more flexibility in controlling output—similar to adjusting RPMs in an internal combustion engine.
3.2 Fusion Without Ignition: A Non-Burning Plasma
Whereas tokamaks chase the elusive dream of plasma ignition (a self-sustaining chain of fusion reactions), Helion’s system operates without this requirement. Each pulse is independent: fusion occurs during a rapid compression event, and then the plasma cools. The process repeats continuously.
This sidesteps one of the hardest physics challenges of steady-state fusion—maintaining plasma stability for long durations, often plagued by turbulence, instabilities, and magnetic reconnection events.
3.3 Energy Recovery: No Steam Required
Most fusion designs propose to extract power by heating a fluid (like water) and driving a turbine, much like a conventional power plant. This results in efficiency losses and bulky infrastructure.
Helion takes a radical departure: its system converts the expanding magnetic field of the post-fusion plasma directly into electricity via Faraday’s Law of Induction.
As the compressed plasma expands, it induces a current in the machine’s surrounding coils. This not only recaptures unused input energy but also converts newly released fusion energy into electricity—all within the same pulse.
This innovation makes Helion’s system more akin to a direct-current generator than a steam plant, and could potentially achieve electrical efficiencies far above 50%.
Fuel Strategy and Direct Electricity Recovery: Helion’s Key Differentiators
4.1 The Fusion Fuel Challenge: Why He-3?
Most fusion systems rely on Deuterium-Tritium (D–T) fuel due to its high fusion reactivity and lower ignition temperature (~100 million °C). However, D–T fusion emits high-energy neutrons, which:
-
Damage reactor materials
-
Activate structural components, producing radioactive waste
-
Require complex tritium breeding blankets
-
Impose significant shielding and safety overhead
Helion avoids this by using a Deuterium–Helium-3 (D–He³) fuel mixture. This reaction releases only charged particles (protons and helium nuclei), meaning:
-
No harmful neutron radiation
-
No radioactive waste
-
Energy can be captured electrically, not thermally
While D–He³ fusion demands much higher temperatures (near 200 million °C), Helion believes its pulsed compression system can meet this threshold more easily than large, steady-state reactors.
“Fusion without neutrons is like fire without smoke. It changes everything about power plant design.” — Helion Tech Brief
4.2 Producing Helium-3 In-House
Helium-3 is rare on Earth and expensive to harvest from external sources (e.g., lunar regolith or dismantled nuclear warheads). Helion mitigates this by producing it in-house:
-
Starts with Deuterium-Deuterium (D–D) fusion pulses
-
One D–D fusion path yields Helium-3 as a byproduct
-
Helion recycles this He-3 into future fuel cycles
This approach allows self-sufficiency, avoiding the supply chain risks of tritium or externally sourced He-3.
4.3 Energy Conversion: From Plasma to Grid
Instead of relying on:
-
Neutron-heated blankets
-
Steam generators
-
Turbines and condensers
Helion’s reactor functions like a plasma transformer:
-
After each fusion pulse, expanding plasma induces current in external coils
-
This electricity is fed directly into the grid or used internally
-
Any unused input energy is also recaptured
This direct recovery model:
-
Increases net system efficiency
-
Reduces cooling and maintenance needs
-
Shrinks plant footprint (no giant cooling towers)
By eliminating the Carnot cycle bottleneck, Helion aims for >50% wall-plug efficiency—unheard of in nuclear energy.
5. Comparative Analysis: Helion vs. Global Fusion Competitors
5.1 Overview of Major Fusion Pathways
We now compare Helion’s MTF approach with:
-
ITER – traditional tokamak, international megaproject
-
CFS – compact tokamak with HTS magnets
-
TAE – beam-driven Field-Reversed Configuration with proton-boron fuel
-
General Fusion – liquid-metal compressed MTF
-
NIF – laser-based inertial confinement
Each uses a distinct fusion philosophy, with trade-offs in size, cost, physics complexity, and commercial readiness.
Company/Project | Fusion Type | Fuel | Status | Timeline | Notable Feature |
---|---|---|---|---|---|
Helion | MTF (Linear FRCs) | D–He³ | 7 prototypes; Polaris (2024) | 50 MW grid by 2028 | Direct electricity recovery |
ITER | Tokamak (Toroidal) | D–T | Under construction | First plasma ~2033 | Longest plasma duration |
CFS | Tokamak (Compact) | D–T | SPARC in dev. | Net energy by 2027 | HTS magnets (20 Tesla) |
TAE | Beam-FRC | p–B¹¹ (future), H now | Copernicus (100 MK) | p–B¹¹ by 2030s | Aneutronic goal |
General Fusion | MTF (Mechanical) | D–T | LM26, FDP in UK | Pilot ~2030s | Liquid metal compression |
NIF | Inertial (Laser) | D–T | Scientific ignition (2022) | No energy recovery | High gain, low repetition |
5.2 Fusion Performance Metrics
Let’s compare key performance indicators (KPIs):
Metric | Helion | ITER | CFS | TAE | General Fusion | NIF |
---|---|---|---|---|---|---|
Reactor Size | Compact | Massive | Medium | Compact | Medium | Fixed lab-scale |
Target Temp. | 200M °C | 100M °C | 100M °C | 3B °C | 150M °C | 100M+ °C |
Fuel Supply Risk | Low (D + bred He-3) | High (Tritium) | High | Medium | Medium | High |
Neutron Output | Low | High | High | None (future) | High | High |
Power Extraction | Direct Electric | Thermal | Thermal | Direct (future) | Thermal | N/A |
Net Gain Achieved | Pending (2024) | No | No | No | No | Scientific gain only |
Repetition Rate | 1/sec (target) | Continuous | Continuous | Steady-state | 1/day (now) | Few/day |
Helion scores particularly well in energy efficiency, fuel safety, and machine simplicity, though it must prove gain and durability under repeated pulses.
Case Study: Helion & Microsoft – The First Fusion Power Purchase Agreement (PPA)
Case Study: Helion & Nucor Steel – Industrial Fusion for Heavy Manufacturing
6.Funding, Timelines, and Technical Milestones
6.1 Fusion Investment Surge
Over the past decade, private fusion investment has shifted from speculative science to serious commercial infrastructure. As of 2025, global private fusion investment has surpassed $6 billion, with Helion Energy among the top recipients. Their fundraising rounds include:
- $0.5 billion in 2021 (Series E)
- Backing from OpenAI CEO Sam Altman, who has invested over $375 million
- Strategic investors: Capricorn Investment Group, Founders Fund, and Y Combinator
By contrast, Commonwealth Fusion Systems (CFS) has raised over $2 billion, primarily for its SPARC tokamak, and TAE Technologies has raised approximately $1.2 billion for its p-B11 beam-driven approach. General Fusion, supported by Jeff Bezos, trails behind at around $450 million. On the public side, ITER commands a massive $22 billion+ government budget, though it is not expected to produce commercial power until the mid-2030s or later.
6.2 Key Technical Milestones by Helion
Helion follows a structured prototype roadmap:
Prototype | Year | Key Achievement |
---|---|---|
Trenta | 2021 | Achieved 100 million °C plasma temperature |
Polaris | 2024 | Targeted to generate first electricity from fusion reaction |
Pilot Plant | 2026–27 | Scaled system testing continuous pulsing at 1Hz |
Commercial 50 MW Plant | 2028 | Contracted power delivery to Microsoft |
Industrial Scale 500 MW | Early 2030s | Partnership with Nucor Steel for co-located fusion plant |
Unlike many competitors, Helion’s development model emphasizes rapid prototyping, low capital intensity per stage, and measurable progress benchmarks.
7. Key Challenges and Strategic Advantages
7.1 Technical Challenges for Helion
Despite impressive milestones, Helion must overcome several critical challenges to succeed:
1. Repetition Rate Scaling
Achieving 1+ pulses per second with precision control, efficient compression, and stable plasma formation is a major engineering hurdle. Reliability over millions of pulses per year must be proven.
2. Plasma-Particle Containment
Although MTF sidesteps long-term magnetic confinement, the fusion pulse must be clean and reproducible. Small misalignments or instabilities in FRC merging can disrupt the fusion gain.
3. Direct Energy Conversion
Capturing electricity from plasma expansion requires precision-tuned inductive systems. Noise, losses, and capacitor wear need extensive testing under real conditions.
4. Helium-3 Production
While D–D fusion can produce He-3, yield rates, purification, and fuel recycling will be mission-critical. Helion must create a reliable on-site fuel loop without external He-3 supply chains.
7.2 Strategic Advantages of Helion’s Approach
1. Aneutronic Advantage
Helion’s use of D–He3 eliminates neutron damage and radioactive waste, drastically reducing costs of shielding, remote handling, and regulatory burdens.
2. Direct Electricity Recovery
By skipping steam turbines, Helion could exceed 50% thermal efficiency and cut capital costs of conventional generation systems.
3. Compact Design & Modular Deployment
Helion’s linear geometry is smaller and more scalable than tokamaks, allowing modular plant deployment in industrial, urban, or off-grid locations.
4. First-Mover Market Credibility
With the first-ever fusion PPA signed, Helion gains both brand authority and a potential revenue stream before competitors.
5. Tech Industry Partnerships
By collaborating with Microsoft and Nucor, Helion positions itself as a fusion solution not only for the grid but for clean data centers and decarbonized industry—a vast, underserved energy segment.
8. Conclusion: Fusion’s Decisive Decade
The 2020s are shaping up to be the decade of commercial fusion—after nearly a century of scientific ambition. As private capital accelerates, fusion startups are beginning to challenge long-standing assumptions, bringing fresh engineering strategies and aggressive go-to-market tactics.
Among them, Helion Energy is arguably the most disruptive. Its Magnetized Target Fusion (MTF) approach challenges the orthodoxy of steady-state tokamaks and inertial lasers. By focusing on rapid pulsing, aneutronic fuels, and direct electricity capture, Helion targets what many others have delayed: a grid-connected, modular, and economically viable fusion system.
However, success is not guaranteed. Helion must still demonstrate:
-
Consistent fusion gain across millions of pulses
-
Safe and scalable He-3 fuel recycling
-
Reliable conversion of plasma energy to electric output
Yet if Polaris succeeds—and the 2028 Microsoft PPA is fulfilled—Helion will not only make history but may also redefine how humanity powers the future.
As governments, investors, and industries turn toward decarbonization, fusion must prove not just that it works, but that it can scale, compete, and sustain. The fusion company that gets there first will shape the global energy landscape for the next hundred years.
And right now, Helion might just be in the lead.